Mucus is Snot a Problem for Bacteria, Which Swarm Through It
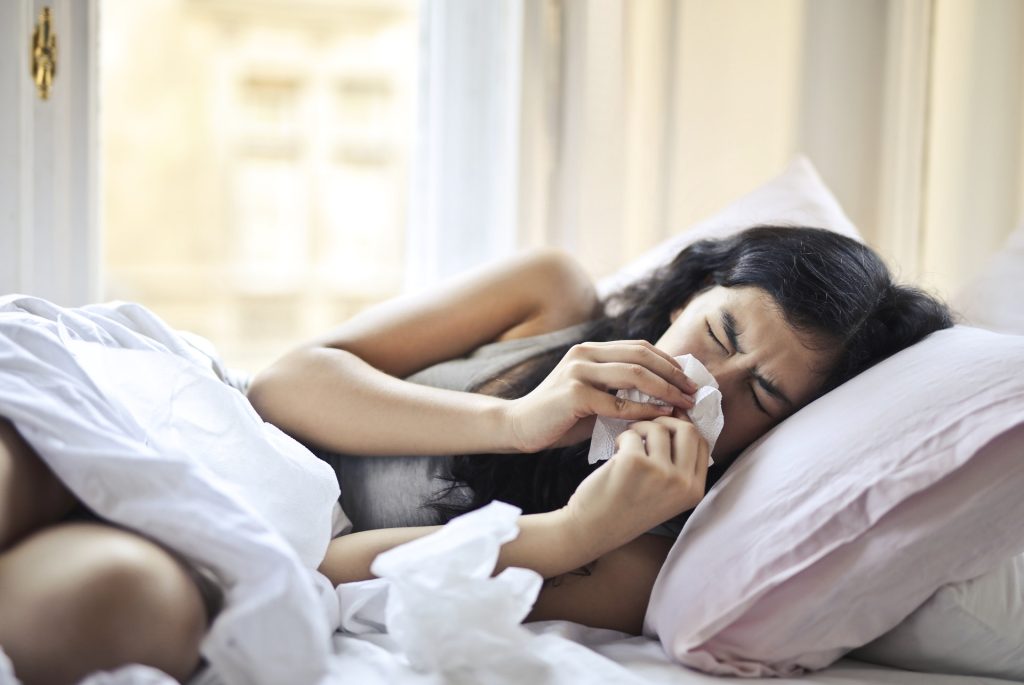
The increase in mucus from sniffles and runny noses is exactly what bacteria use to mount a coordinated attack on the immune system, according to a new study from researchers at Penn State. The team found that the thicker the mucus, the better the bacteria are able to swarm. The findings could inform treatments to control the spread of bacteria.
The study, recently published in the journal PNAS Nexus, demonstrates how bacteria use mucus to enhance their ability to self-organise and possibly drive infection.
The experiments, performed using synthetic pig stomach mucus, natural cow cervical mucus and a water-soluble polymer compound called polyvidone, revealed that bacteria coordinate movement better in thick mucus than in watery substances.
According to the researchers, the findings provide insight into how bacteria colonise mucus and mucosal surfaces, and also show how mucus enhances bacterial collective motion, or swarming, which may increase antibiotic resistance of bacterial colonies.
“To the best of our knowledge, our study is the first demonstration of bacteria collectively swimming in mucus,” said Igor Aronson, Huck Chair Professor of Biomedical Engineering, of Chemistry and of Mathematics at Penn State and corresponding author on the paper.
“We have shown that mucus, unlike liquids of similar consistency, enhances the collective behaviour.”
Mucus is essential for many biological functions, explained Aronson. It lines the surfaces of cells and tissues and protects against pathogens such as bacteria, fungi and viruses. But it is also the host material for bacteria-born infections, including sexually transmitted and gastric diseases.
A better understanding of how bacteria swarm in mucus could lead to new strategies to combat infections and the growing problem of antibiotic resistance, according to Aronson.
“Our findings demonstrate how mucus consistency affects random motion of individual bacteria and influences their transition to coordinated, collective motion of large bacterial groups,” Aronson said.
“There are studies demonstrating that collective motion or swarming of bacteria enhances the ability of bacterial colonies to fend off the effect of antibiotics. The onset of collective behaviour studied in our work is directly related to swarming.”
Mucus is a notoriously challenging substance to study because it exhibits both liquid-like and solid-like properties, Aronson explained.
Liquids are typically described by their level of viscosity, how thick or thin the liquid is, and solids are described by their elasticity, how much force it can take before breaking. Mucus, a viscoelastic fluid, behaves as both a liquid and solid.
To better understand how mucus becomes infected, the team used microscopic imaging techniques to observe the collective motion of the concentrated bacteria Bacillus subtilis in synthetic pig stomach mucus and natural cow cervical mucus, which for this purpose are analogous to human mucus.
They compared those results with observations of Bacillus subtilis moving in a water-soluble polymer polyvidone at a wide range of concentrations, from high to low levels of polyvidone.
The researchers also compared their experimental results to a computational model for bacterial collective motion in viscoelastic fluids like mucus.
The team found that mucus consistency profoundly affects the collective behaviour of bacteria: the thicker the mucus, the more likely the bacteria would exhibit collective movement, forming a coordinated swarm.
“We were able to show how the viscoelasticity in mucus enhances bacterial organisation, which in turn leads to coherently moving bacterial groups that cause infection,” Aronson said.
“Our results reveal that the levels of elasticity and viscosity in mucus are a main driver in how bacterial communities organize themselves, which can provide insight into how we can control and prevent bacterial invasion in mucus.”
Aronson explained that the team expects human mucus to exhibit similar physical properties, meaning their findings are also relevant for human health.
“Our results have implications for human and animal health. We’re showing that mucus viscoelasticity can enhance large-scale collective motion of bacteria, which may accelerate how quickly bacteria penetrate mucus protective barrier and infect internal tissues.”
Source: Penn State